Holography - News & Articles
Color holography: its history, state-of-the-art and future
Presented at HOLO 05, Varna, Bugaria, May, 2005
reprinted with permission of the author
Hans I. Bjelkhagen
North East Wales Institute of Higher Education, Dept. of Science, Wrexham LL11 2AW, UK
OpTIC Technium, Centre for Modern Optics, St Asaph Business Park, St Asaph LL17 0JD, UK
ABSTRACT
The first methods for recording color holograms were established in the early 1960s. Leith and Upatnieks proposed multicolor wavefront reconstruction and Denisyuk introduced the single-beam reflection holography technique which is most suitable for recording color holograms today. Reviewed is the history of color holography highlighting important milestones. The current state-of-the-art of color holography is presented including the recording techniques using red, green and blue laser wavelengths. The laser wavelength selection issue is presented using computer simulation, showing that more than three wavelengths may be needed for accurate color rendition in holograms. The recording material is a key factor very important for creating high-quality color holograms. Covered are both the demand on the material and suitable products currently on the market. The future of color holography is highly dependent on the availability of improved panchromatic recording materials and suitable light sources for displaying the holograms. Small laser diodes as well as powerful white LEDs and OLEDs with very limited source diameters are important for color holography to become an important 3D display medium.
Keywords: color holography, color reproduction
1. INTRODUCTION
After more than 40 years since the appearance of the first
monochromatic laser-recorded holograms the possibilities of recording
high-quality holograms in true colors are now a reality. In this paper we are
only concerned about color holograms recorded of objects, which after
processing and upon reconstruction, display the colors of the recorded object as
accurate as possible. Although special techniques allow for the production of
display holograms exhibiting different colors, in most cases the colors
displayed in these holograms are not the true, original colors of the recorded
object. These holograms are often referred to as pseudo- or multicolor
holograms. There exist methods for creating colors that give an impression of
true color in the finished image in a holographic stereogram made from multiple
sets of color-separated photographs. By using the rainbow technique it is
possible to produce holograms with correct colors, however, only possible to
view at a certain position.
The holograms recorded of objects with the correct color
image displayed are referred to as color holograms. However, colors of
the objects we normally see around are sometimes impossible to record
holographically since holograms can only reproduce colors of objects created by
the laser light scattered. Colors we see are often the result of
fluorescence which cannot be recorded in a hologram. For example, some dyed and
plastic objects achieve their bright, saturated colors by fluorescence. This
limitation in color holography does not seem to be very dramatic.
To be able to record high-quality color reflection holograms
it is necessary to use extremely low scattering recording materials, which
means, for example, the use of ultra-high resolution silver-halide emulsions.1
This type of material has the advantage of higher sensitivity compared to
photopolymer or dichromated gelatin (DCG) materials which are alternative
materials for color holography. Ultra-high resolution
silver-halide emulsions for monochrome holography have been manufactured in
Russia for many years and currently these emulsions are panchromatic. In this
paper we refer
to the ultra-high-resolution holographic emulsions (grain
size 10 to 20 nm) as "Russian" emulsions since they were first made in Russia.
Denisyuk and Protas2 were able to slow down grain growth during
emulsification by increasing the
hansholo@aol.com phone: +44 1745 535130; fax: +44 1745 535101;
www.optictechnium.com
number of growth centers and introducing special growth
inhibitors. The best Russian emulsion is probably the one achieved by Kirillov3.
In this case, grain growth was hampered by the fact that in the emulsification
process a highly diluted solution was used and the emulsion concentration was
increased by applying the method of gradual freezing and thawing. The highest
resolution holographic materials used for monochrome holography and previously
manufactured by Agfa, Ilford, and Kodak had a grain size of 35 nm to 50 nm.
These products are no longer on the market; however, they would not be suitable
for color holography today.
2. HISTORY OF COLOR HOLOGRAPHY
The first methods for recording color holograms were
established in the early 1960s. Leith and Upatnieks proposed multicolor
wavefront reconstruction in one of their early papers.4 Mandel5
pointed out that it might be possible to record color holograms in a more
straightforward way, using a polychromatic laser and an off-axis setup. Lohmann6
introduced polarization as an extension of the suggested technique. These first
methods concerned mainly transmission holograms recorded with three
different wavelengths from a laser or lasers, combined with different reference
directions to avoid cross-talk. The color hologram was then reconstructed using
the original laser wavelengths from the corresponding reference directions.
Color holograms of a reasonably high quality could be made this way, but the
complicated and expensive reconstruction setup prevented this technique from
becoming popular. The first transmission color hologram shown in Fig. 1 was made
by Pennington and Lin.7 They used the 15
μm thick Kodak 649-F emulsion with a
spectral bandwidth of about 10 nm. This narrow bandwidth eliminated, in
principle, cross-talk between the two colors (633 nm and 488
nm) at the reconstruction.
The Lippmann color technique is very interesting for color
recordings using reflection holograms. Lin et al.8 made
the first color reflection hologram that could be reconstructed in white light.
They recorded a reflection hologram of a color slide illuminated with two
wavelengths (633 nm and 488 nm). The material used here was the Kodak 649-F
plate which was processed without fixing in order to avoid shrinkage. This
technique, using reflection holography and white-light reconstruction technique,
seems to be the most promising one as regards the actual recording of
color holograms and will be further discussed later on. However, the three-beam
transmission technique might eventually become equally applicable, since
inexpensive multicolor semiconductor lasers exist on the market today. Other
early papers on color holography published in the sixties are found in Refs
9-16. An early color reflection hologram by Lin and LoBianco15 is
shown in Fig. 2.
Relatively few improvements in color holography were made
during the seventies and eighties, although some important papers on color
holography were published.17-28 During the next decade and until
today new and improved techniques have been introduced.29-51 A review
of various transmission and reflection techniques for color holography can be
found in a 1983 publication by Hariharan.23 In regard to reflection
color holography, an early extensive contribution was made by Hubel and Solymar.32
Bjelkhagen et al.36 included an extensive bibliography on
color holography in a paper on color holograms recorded in a single layer silver
halide emulsion.
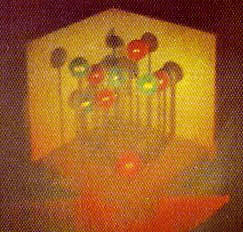
Fig. 1. First
color hologram, Fig. 2. A 1967 reflection color hologram
recorded 1965
Color reflection holography presents no problems as regards
the geometry of the recording setup, but the final result is highly dependent on
the recording material used and the processing techniques applied. The
single-beam Denisyuk recording scheme has produced the best results so far.
Color holograms have been recorded in single-layer silver-halide emulsions, or
in two separate silver-halide emulsions in a sandwich. Color holograms in DCG
emulsion and a DCG emulsion in combination with a silver-halide emulsion in a
sandwich have also been demonstrated. Even three-layer emulsions for color
holography have been manufactured.31
Kubota and Ose20 demonstrated that a good color
reflection hologram could be recorded in DCG which gives high efficiency and
low-noise blue reconstruction. The most successful sandwich recording technique
was demonstrated in 1986 by Kubota28 who used a dichromated gelatin
plate for the green (515 nm) and the blue (488 nm) components, and an Agfa 8E75
plate for the red (633 nm) component of the image. This hologram is shown in
Fig. 3. Since the DCG plate is completely transparent in red light, the
silver-halide plate (containing the red image) is mounted behind the DCG-plate
in relation to the observer.
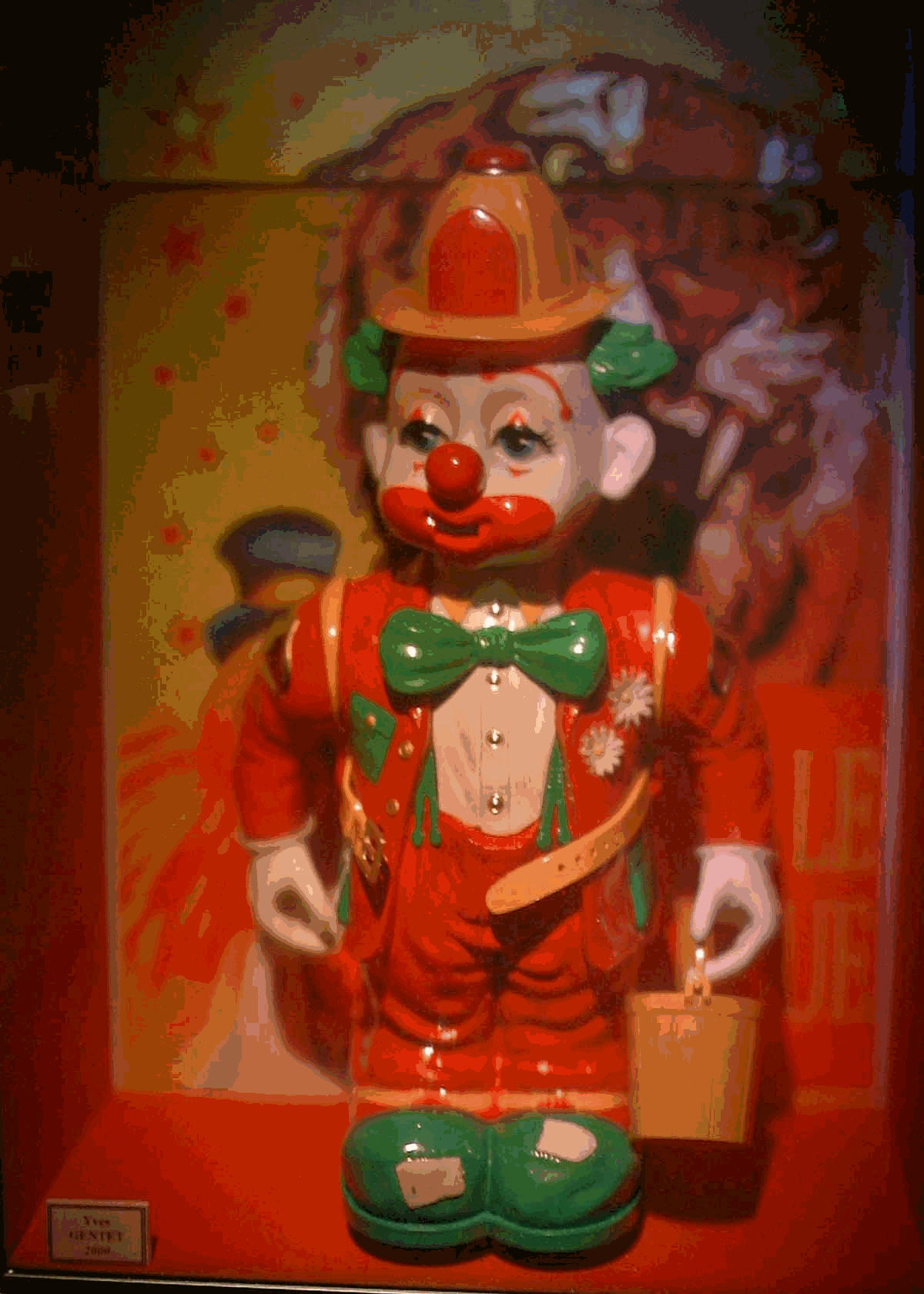
Fig. 3.Kubota’s Fig. 4. Hologram in a single-
Fig. 5. Hologram in
sandwich hologram. layer Slavich emulsion Gentet’s
Ultimate emulsion
When improved panchromatic silver halide emulsions
manufactured by Slavich were introduced Bjelkhagen and Vukičević35
and Bjelkhagen et al.36demonstrated that high-quality
holograms could be recorded in a single layer emulsion shown in Fig. 4. More
recently Gentet45 was able to record very high-quality holograms in
an ultra-fine-grain silver halide Ultimate emulsion as illustrated in
Fig.5. The possibility to record a color hologram in photopolymer recording
materials was first demonstrated by Kurtzner and Haines.18

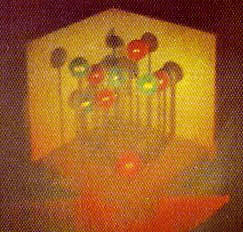
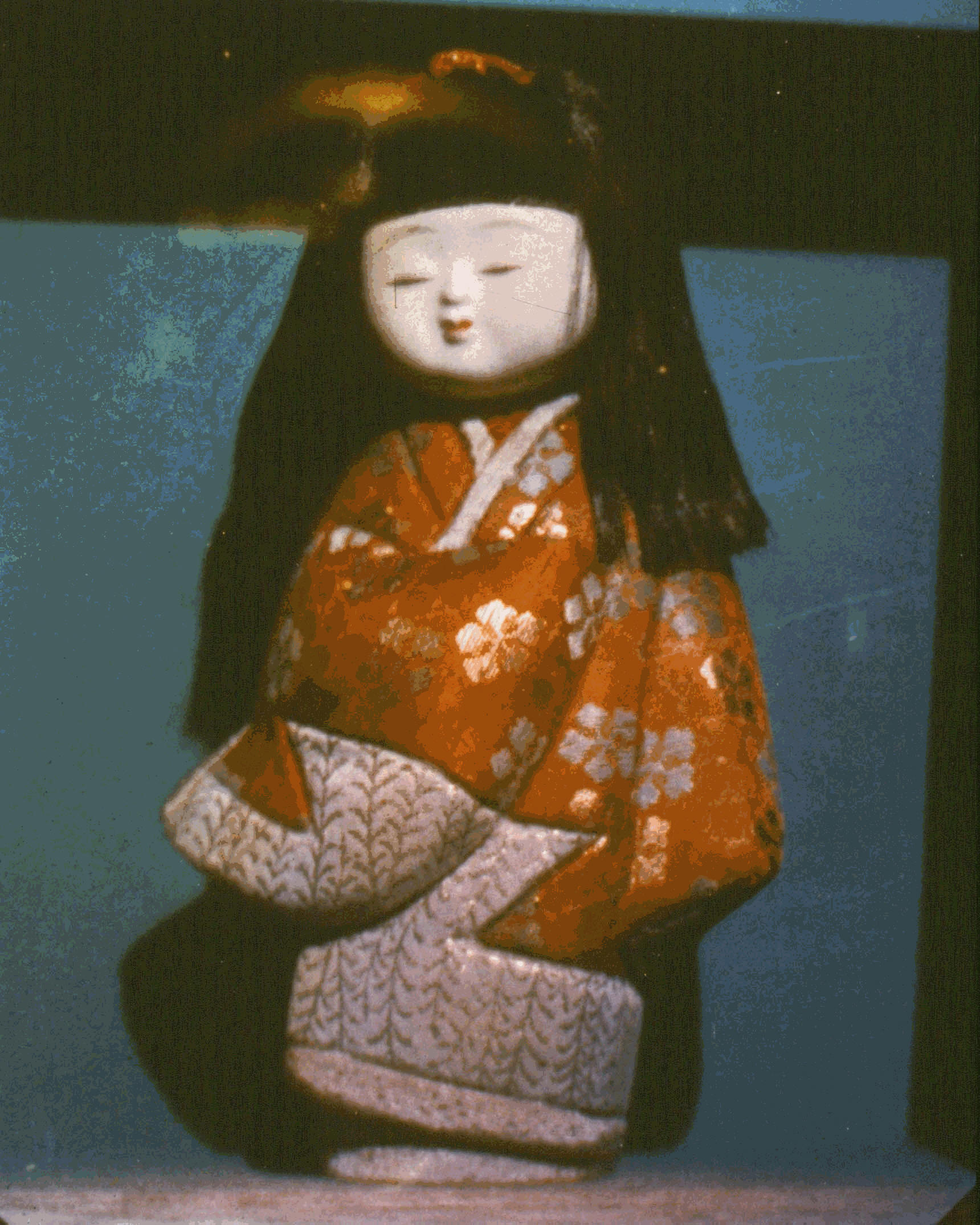

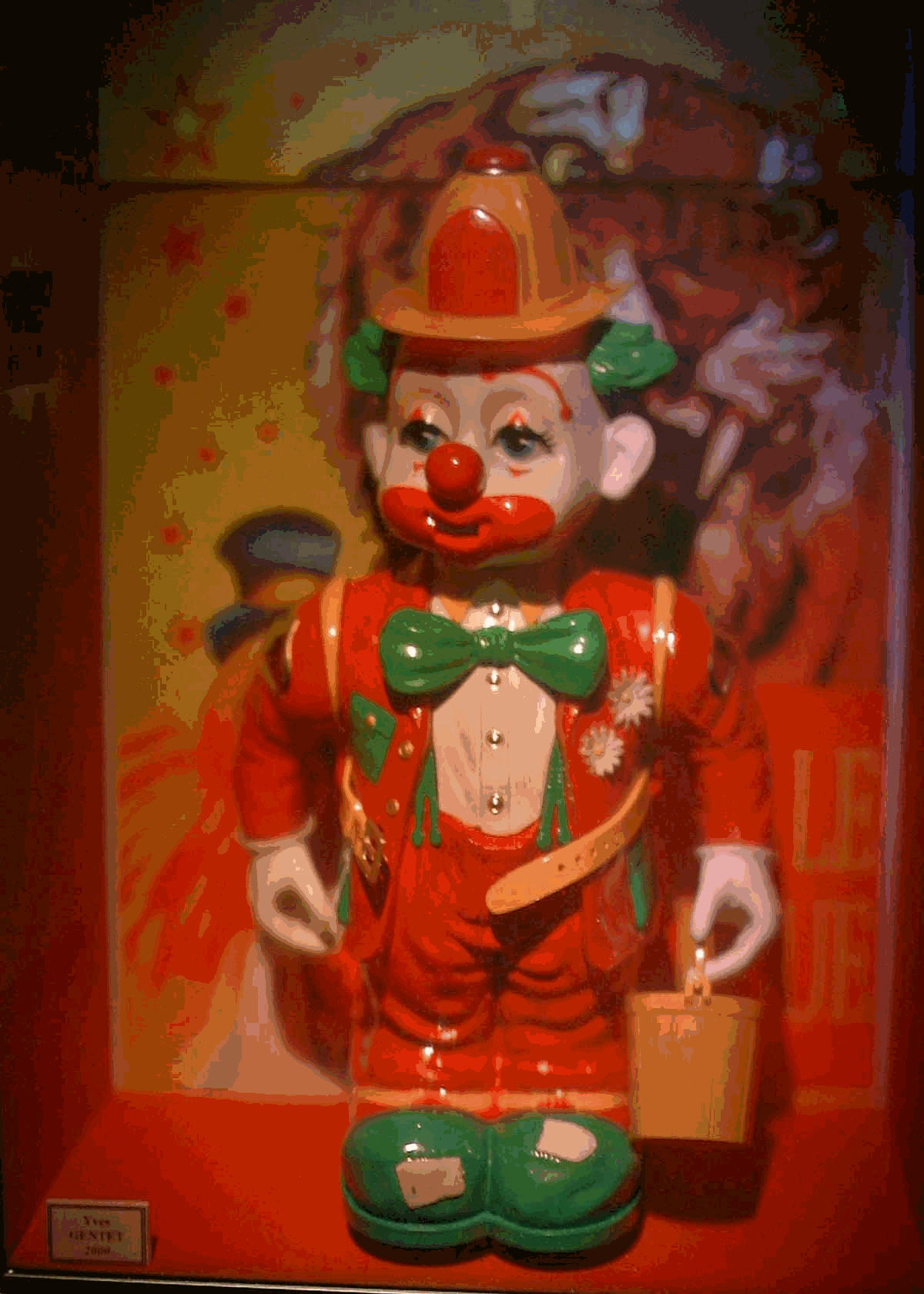
3. Color Holography
3.1 Laser wavelengths for recording color holograms
The problem of choosing optimal primary laser wavelengths for color holography is illustrated in the 1976 CIE diagram with indication of suitable laser wavelengths for color holograms shown in Fig. 6. The diagram is useful for predicting the colors that can be matched by additive mixing of a set of primary laser wavelengths. After the three primary spectral colors have been selected, a triangle is made by joining the three points corresponding to the spectral colors of the diagram. The colors within the area covered by the triangle correspond to all the colors that can be produced by appropriate mixing of the chosen spectral colors. It may seem that the main aim of choosing the recording wavelengths for color holograms would be to cover as large an area of the chromaticity diagram as possible. However, there are many other considerations to be taken into account when choosing the wavelengths for color holograms. One important question is whether three wavelengths are really sufficient for color holography.
Fig. 6. The 1976 CIE uniform scales chromaticity diagram shows the gamut of surface colors and positions of common laser wavelengths including optimal color-recording laser wavelengths
Hubel and Solymar32 gave a quantitative definition of a color hologram: "A holographic technique is said to reproduce 'true' colors if the average vector length of a standard set of colored surfaces is less than 0.015 chromaticity coordinate units, and the gamut area obtained by these surfaces is within 40% of the reference gamut. Average vector length and gamut area should both be computed using a suitable white light standard reference illuminant."
The wavelength selection problem for color holography has been treated in many publications, for example, by Peercy and Hesselink,34 Kubota et al.46 and recently by Mirlis et al.52 Peercy and Hesselink,34 discussed wavelength selection by investigating the sampling nature of the holographic process. During the recording of a color hologram the chosen wavelengths point sample the surface-reflectance functions of the object. This sampling on color perception can be investigated by the tristimulus value of points in the reconstructed hologram which is mathematically equivalent to integral approximations for the tristimulus integrals. They used both Gaussian quadrature and Riemann summation for the approximation of the tristimulus integrals. In the first case they found the wavelengths to be 437, 547, and 665 nm. In the second case the wavelengths are 475, 550, and 625 nm. According to Peercy and Hesselink, the sampling approach indicates that three monochromatic sources are almost always insufficient to preserve all of the object's spectral information accurately. They claim that four or five laser wavelengths may be required.
Kubota et al.46 presented a theoretical analysis of color holography based on four recording wavelengths. Using the 1976 CIE chromaticity diagram, and by minimizing the distance between the selected object points in the diagram and the corresponding reconstructed image points, they were able to obtain four optimal laser wavelengths. The calculation was based on the nonlinear least square method. For the reproduction of nineteen selected color patches of the Macbeth ColorChecker7 the following four wavelengths were obtained:
λ1= 459.1 nm, λ2 = 515.2 nm, λ3 = 585.0 nm, and λ4 = 663.2 nm.
Mirlis et al.52 employed a computer program which takes as an input the number of sources of monochromatic light to be employed during the recording and the wavelength of each source. As an output it gives the average total error between all the Macbeth color patches as well as the errors for each individual patch, expressed in x, y CIE chromaticity units. It is possible to choose any number of laser sources between 3 and 300, with any wavelengths within the visible spectrum that is assumed to be from 400 nm to 700 nm. The first set of simulations was performed in an attempt to answer the question of how many laser sources are needed in order to achieve a desired color rendition. In order to answer this question the algorithm was programmed to run over all possible combinations of wavelengths within the visible spectrum performing simulations employing from three up to seven laser sources of monochromatic light. The best selections (smaller average error) were then plotted on a graph illustrated in Fig.7. Mirlis et al52 obtained the following optimal four laser wavelengths:
λ1 = 479 nm, λ2 = 518 nm, λ3 = 571 nm, and λ4 = 620 nm.
Fig. 7. Total average error vs number of laser wavelengths
Referring to Fig. 7 the important part is from three to seven laser sources where the average error reduces significantly. Furthermore, as the number of laser sources increases to more than five, the error changes only marginally. As a result, we can assume that four to five laser sources are a sufficient number to assure minimal error. The choice between four or five lasers depends on the nature of the application and is a trade-off between cost and quality. The best selections from three and four lasers are illustrated in Fig. 8 showing individuals errors for each Macbeth ColorChecker® color patches. A significant improvement is achieved by using four recording wavelengths.
Three optimal laser wavelengths
Four
optimal laser wavelengths
Note that the Y-axis scale factor is different in the figures above.
Fig. 8 Error graphs for the three and four laser wavelengths simulations, where the error for each color patch of the target is expressed in CIE x,y units. At the bottom the GretagMacbeth ColorChecker® target is illustrated. The grey-scale colors of the test target were not included in the simulations
Fig. 9. The setup for recording color holograms
3.2. Recording of color holograms
Up until now most color holograms have been recorded using three laser wavelengths. Almost exclusively all color holograms recorded so far are produced with cw lasers. However progress in pulsed color lasers may change the situation in the near future. Geola is manufacturing a pulsed three-color laser in Lithuania.53 Employing such a laser, dynamic events, as well as portraits, can be recorded in a holographic form. However, the pulsed laser output energy is limited and improvements are needed.
3.3. Setup for recording color holograms
A typical reflection hologram recording setup is illustrated in Fig 9. For most display purposes, the very large field of view obtainable in a single-beam Denisyuk hologram is very attractive. Therefore such a recording scheme is selected. The different laser beams necessary for the exposure of the object pass through the same beam expander and spatial filter. In the Denisyuk arrangement, the object is illuminated through the recording holographic plate. The light reflected from the object constitutes the object beam of the hologram. The reference beam is formed by the three expanded laser beams. This "white" laser beam illuminates both the holographic plate and the object itself through the plate. Each of the three primary laser wavelengths forms its individual interference pattern in the emulsion, all of which are recorded simultaneously during the exposure. In this way, three holographic images (a red, a green, and a blue image) are superimposed upon one another in the emulsion.
Three primary laser wavelengths are employed for the recording: for example, 476 nm, provided by an argon ion laser, 532 nm, provided by a cw frequency-doubled Nd:YAG laser, and 647 nm, provided by a krypton ion laser. Two dichroic filters are used for the combining of the three laser beams. The "white" laser beam goes through a spatial filter, illuminating the object through the holographic plate. By using dichroic filter beam combiners, simultaneous exposure recording can be performed. This makes it possible to control independently the RGB ratio and the overall exposure energy in the emulsion. The RGB ratio can be varied by individually changing the output power of the lasers, while the overall exposure energy is controlled solely by the exposure time.
3.4 Recording materials
The main key to succeed in making color holograms is to have a suitable recording material. To be able to record high-quality color reflection holograms it is necessary to use extremely low light-scattering panchromatic recording materials. Currently, the main materials are the silver halide emulsions and the photopolymer materials. There are several problems associated with the recording color reflection holograms. Light scattering occurring in the blue part of the spectrum of many materials exclude them from being used, e.g., many holographic silver halide emulsions. Another problem is that multiple exposures of a single emulsion may reduce the diffraction efficiency of each individual recording. A third problem is the shrinkage of the material that often takes place during processing, causing a wavelength shift in the reconstructed image. White-light-illuminated reflection holograms normally show an increased bandwidth upon reconstruction, thus affecting the color rendition. In addition to recording material problems, the selection of laser wavelengths is important to obtain the best possible color rendition of the object.
3.4.1. Silver halide materials
There are very few materials of the silver halide type on the market suitable for color holography. What is needed here is a panchromatic ultra-fine-grain silver halide emulsions (grain size about 10 nm or less). Currently the main commercial producer of such a material is the Slavich company54 in Russia. Some characteristics of the Slavich PFG-03c emulsion are presented in Table 1.
Silver halide material PFG-03C
Emulsion thickness 7 μm
Grain size 12 - 20 nm
Resolution ~10000 lp/mm
Blue sensitivity ~1.0 - 1.5× 10-3 J/cm2
Green sensitivity ~1.2 - 1.6× 10-3 J/cm2
Red sensitivity ~0.8 - 1.2× 10-3 J/cm2
Color sensitivity peaked at: 633 nm, and 530 nm
- - - - - - - - - - - - - - - - - - - - - - - - - - - - - - - - - - - - - - - - - -
Table 1. Characteristics of the Slavich panchromatic emulsion
There are a few other companies producing ultra-fine-grain emulsions for holography, but, so far, only monochromatic versions. In France, Gentet is running a small-scale manufacturing facility to produce a new panchromatic silver halide emulsion with extremely fine grains.45 The material is called the Ultimate emulsion. However, Gentet's color emulsion is mainly used for his own recording of color holograms.
The processing of silver halide emulsions is more difficult and critical than for monochrome reflection holograms. Emulsion shrinkage and other emulsion distortions caused by the active solutions used for the processing must be avoided. More details on processing color holograms are provided in Ref. 36.
A recorded color hologram of a test object with the CIE 1931 test target is illustrated in Fig.10. The color balance for the recording of a color hologram must be adjusted with what type of spotlight that is going to be used for the display of the finished hologram in mind. Figure 11 shows a typical normalized spectrum obtained from a white area of the color test target hologram. One should note the high diffraction efficiency in blue, needed to compensate for the rather low blue light emission of the halogen spotlight. The noise level, mainly in the blue part of the spectrum, is visible and low. The three peaks are exactly at the recording wavelengths; i.e., 647, 532, and 476 nm.
Fig. 10. CIE test target hologram Fig. 11. Color spectrum from white area of the test target
In Fig 12 the setup for the recording of an 8" by 10" color hologram of a painted Russian Egg is shown. Note that the object is positioned upside-down on the table. In this way an over-head reference beam is easily provided for the final hologram. The unexposed holographic glass plate is positioned on a three-point mount in front of the object. A photo of the recorded hologram is shown in Fig.13. Note that the white background behind the egg is reproduced in a blue-green color. This was caused by using, in this case, a HeNe laser for the red component. The HeNe laser has very limited coherence length, which means that it could not record the white background which was too far behind the egg. Only the blue and green wavelengths could record the background. This problem is important to be aware of when recording color holograms. However, in this hologram the blue background may be nice but it is not a correctly recorded color hologram.
Fig. 12. Recording setup Fig. 13. Russian egg hologram
Variation in coherence length or any laser beam disturbances during recording of a color hologram in any of the recording laser wavelengths will result in problems with recording white areas of the object and, of course, incorrect rendering of other colors as well. A very stable recording setup is necessary as well as lasers with extremely stable output beams to create perfect color holograms.
3.2.2. Photopolymer materials
The panchromatic photopolymer material from DuPont is an alternative recording material for color holograms.37,38 Although, being less sensitive than the ultrafine-grain silver halide emulsions, it has its special advantages of easy handling and dry processing (only UV-curing and baking.) The color photopolymer material needs an overall exposure of about 10 mJ/cm2. After the exposure is finished, the film has to be exposed to strong white or UV light; about 100 mJ/cm2 exposure at 350-380 nm. After that, the hologram is put in an oven at a temperature of 120oC for two hours in order to increase the brightness of the image. Recently DuPont announced that their materials will no longer be on the market. DuPont will be using the materials for their own production of holograms and HOEs. Only special customers working in the field of optical security may still be able to obtain DuPont photopolymer materials, for example, DAI Nippon Printing Co., Ltd. (DNP) in Japan.47 In 1998, as the first company in the world and still the only one, DNP launched the mass-produced color holograms recorded in DuPont's panchromatic photopolymer material.47 The mass-produced decorative holograms called TRUE IMAGETM and the security color holograms are marketed under the name of SECURE IMAGETM.
4. THE FUTURE OF COLOR HOLOGRAPHY
The recording of large-format color holograms in ultra-fine-grain single-layer silver-halide emulsions is the most promising way forward. With an optimal choice of the amount and position of the recording laser wavelengths within the spectrum, good color rendering can be achieved on such materials. Problems connected with color saturation still remain to be solved. The development process can be further improved in order to avoid desaturation caused by non-uniform development. In addition, more accurate color rendering analysis of holographic recordings is recommended using, e.g., the Macbeth Color Checker chart and colorimetric evaluation as well as light scattering noise measurements. Manufacturing emulsions with a grain size less than 10 nm and at least four recording laser wavelengths will soon make it possible to record a holographic image that would not be possible to distinguish from the object itself.
The virtual color image behind a holographic plate represents the most realistic-looking image of an object that can be recorded today. The extensive field of view adds to the illusion of beholding a real object rather than an image of it. The wavefront reconstruction process recreates accurately the laser wavelengths scattered off the object during the recording of the color hologram. When perfected, this 3D imaging technique has many obvious applications, in particular, in displaying unique and expensive artifacts. There are also many potential commercial applications of color holograms. It may sound strange, but actually, color holography may become an important reproduction technique for 2D objects as well, such as, e.g. oil paintings.48 Holographic reproductions provide extremely realistic-looking images, showing the texture details such as brush strokes and the painter's signature. In addition, they will not fade or change color even if they are continuously on display. This fact is of importance from an archival point of view as well.
Today, it is technologically possible to record and replay acoustic waves with very high fidelity. Hopefully, holographic techniques will be able to offer the same possibility in the field of optical waves, wavefront storage, and reconstruction. Computer-generated color holographic images would make it possible to display extremely realistic full-parallax 3D color images which could be applied in rapid product prototyping, as well as in other applications in 3D visualization and for 3D art.
REFERENCES
1. H.I. Bjelkhagen, Silver Halide Recording Materials for Holography and Their Processing, Springer Series in Optical Sciences, Vol. 66. Springer-Verlag, Heidelberg, New York 1993.
2. Yu.N. Denisyuk, and I.R. Protas, Improved Lippmann photographic plates for recording stationary light waves, Opt. Spectrosc. (USSR) 14, 381-383 (1963).
3. N.V. Kirillov, V.L. Vasilieva,, and Zielikman, Preparation of concentrated photographic emulsions by means of their successive freezing and thawing (in Russian). Zh. Nauchn. Prikl. Fotogr. Kinematogr. 15, 441-443 (1970).
4. E.N. Leith, and J. Upatnieks, Wavefront reconstruction with diffused illumination and three-dimensional objects, J. Opt. Soc. Am. 54, 1295-1301 (1964).
5. L. Mandel, Color imagery by wavefront reconstruction, J. Opt. Soc. Am. 55, 1697-1998 (1965).
6. A.W. Lohmann, Reconstruction of vectorial wavefronts, Appl. Opt. 4, 1667-1668 (1965).
7. K.S. Pennington, and L.H. Lin, Multicolor wavefront reconstruction, Appl. Phys. Lett. 7, 56-57 (1965).
8. L.H. Lin, K.S. Pennington, G.W. Stroke, and A.E. Labeyrie, Multicolor holographic image reconstruction with white-light illumination, Bell Syst. Tech. J. 45, 659-661 (1966).
9.A.A. Friesem, and R.J. Fedorowicz, Recent advances in multicolor wavefront reconstruction, Appl. Opt. 5, 1085-1086 (1966).
10..J. Upatnieks, J. Marks, and R. Fedorowicz, Color holograms for white light reconstruction, Appl. Phys. Lett. 8, 286-287 (1966).
11. G.W. Stroke, and R.G. Zech, White-light reconstruction of color images from black-and-white volume holograms recorded on sheet film, Appl. Phys. Lett. 9, 215-217 (1966).
12. E. Marom, Color imagery by wavefront reconstruction, J. Opt. Soc. Am. 57, 101-102 (1967).
13. A.A. Friesem, and R.J. Fedorowicz, Multicolor wavefront reconstruction, Appl. Opt. 6, 529-536 (1967).
14. R.J. Collier, and K.S. Pennington, Multicolor imaging from holograms formed on two-dimensional media, Appl. Opt. 6, 1091-1095 (1967).
15.reconstructed holograms, Appl. Opt. 6, 1255-1258 (1967).
16. A.A. Friesem, and R.J. Fedorowicz, Multicolor holography, in Holography, ed. by T.H. Jeong and J.E. Ludman. Proc. SPIE 15, 41-48 (1968).
17. A.A. Friesem, and J.L. Walker, Thick absorption recording media in holography, Appl. Opt. 9, 201-214 (1970).
18. E.T. Kurtzner, and K.A. Haines, Multicolor images with volume photopolymer holograms, Appl. Opt. 10, 2194-2195 (1971).
19. P. Hariharan, W.H. Steel, and Z.S. Hegedus, Multicolor holographic imaging with a white-light source, Opt. Lett. 1, 8-9 (1977).
20. T. Kubota, and T. Ose, Lippmann color holograms recorded in methylene-blue-sensitized dichromated gelatin, Opt. Lett. 4, 289-201 (1979).
21. P. Hariharan, Improved techniques for multicolor reflection holograms, J. Optics 11, 53-55 (1980).
22. G.A. Sobolev, and O.B. Serov, Recording color reflection holograms, Sov. Tech. Phys. Lett. 6, 314-315 (1980).
23. P. Hariharan: Colour holography, in Progress in Optics 20, 263-324 (North-Holland, Amsterdam 1983).
24. M.K. Shevtsov, Diffraction efficiency of phase holograms for exposure superposition, Sov. J. Opt. Technol. 52, 1-3 (1985).
25. F.T.S. Yu and G. Gerhart, White light transmission color holography: a review. Opt. Eng. 24, 812-819 (1985).
26.V.P. Smaev, V.Z. Bryskin, E.M. Znamenskaya, A.M. Kursakova, and I.B. Shakhova, Features of the recording of holograms on a two-layer photographic material, Sov. J. Opt. Technol. 53, 287-290 (1986).
27.V. Sainov, S. Sainov, and H. Bjelkhagen, Color reflection holography, in Practical Holography, ed. by T.H. Jeong, and J.E. Ludman. Proc. SPIE 615, 88-91 (1986).
28. T. Kubota, Recording of high quality color holograms, Appl. Opt. 25, 4141-4145 (1986).
29. T. Kubota, and M. Nishimura, Recording and demonstration of cultural assets by color holography (I) - Analysis for the optimum color reproduction, J. Soc. Photogr. Sci. Tech. Jpn. 53, 291-296 (1990).
30. T. Kubota, M. Nishimura, Recording and demonstration of cultural assets by color holography (II) - Recording method of hologram for optimizing the color reproduction. J. Soc. Photogr. Sci. Tech. Jpn. 53, 297-302 (1990).
31. V.P. Smaev, A.D. Galpern, and Yu. A. Vavilova, Three-layer material for the registration of coloured holograms, in Three-Dimensional Holography: Science, Culture, Education, ed. by T.H. Jeong, and V.B. Markov. Proc. SPIE 1238, 311-315 (1991).
32. P.M. Hubel and L. Solymar, Color reflection holography: theory and experiment, Appl. Opt. 30, 4190-4203 (1991).
33. M. Kawabata, A. Sato, I. Sumiyoshi, and T. Kubota, Photopolymer system and its application to a color hologram, Appl. Opt. 33, 2152-2156 (1994).
34. M.S. Peercy and L. Hesselink, Wavelength selection for true-color holography, Appl. Opt. 33, 6811-6817 (1994).
35. H.I. Bjelkhagen and D. Vuki… eviƒ : Lippmann color holography in a single-layer silver-halide emulsion, in Fifth Int'l Symposium on Display Holography, ed. by T.H. Jeong. Proc. SPIE 2333, 34-48 (1995).
36. H. I. Bjelkhagen, T. H. Jeong, and D. Vuki… evi‡ , Color reflection holograms recorded in a ultrahigh-resolution single-layer silver halide emulsion, J. Imaging Sci. Technol. 40, 134-146 (1996).
37. W.J. Gambogi, W.K. Smothers, K.W. Steijn, S.H. Stevenson, and A.M. Weber, Color holography using DuPont holographic recording film, in Holographic Materials, ed. by J. Trout. Proc. SPIE 2405, 62-73 (1995)
38 T.J. Trout, W.J. Gambogi, and S.H. Stevenson, Photopolymer materials for color holography, in Applications of Optical Holography, ed. by T. Honda. Proc. SPIE 2577, 94-105 (1995).
39. H.I. Bjelkhagen and Q. Huang, Large-format color holograms recorded on ultra-high resolution silver-halide emulsions, in Holography and Optical Information Processing (ICHOIP'96), ed. by G. Mu, G. Jin, T. Sincerbox, J. Chen, A. He, D. Hsu. Proc. SPIE 2866, 227-234 (1996).
40. M. Iwasaki, H. Shindo, T. Tanaka, and T. Kubota, Spectral evaluation of laboratory-made silver halide emulsions for color holography. J. Imaging Sci. Technol. 41, 457-467 (1997).
41 T. Jeong, H.I. Bjelkhagen, and L. Spoto, Holographic interferometry with multiple wavelengths. Appl. Opt. 36, 3686-3688, (1997).
42. J. Hartong, J. Sadi, M. Torzynski and D. Vukicevic, Speckle phase averaging in high-resolution color holography, J. Opt. Soc. Am. A 14, 405-410 (1997).
43. M. Iwasaki and T. Kubota, Ultra-fine-grain silver halide emulsions for color holography: preparation and spectral characterization 41, in Sixth Int'l Symposium on Display Holography, ed. by T.H. Jeong, H.I. Bjelkhagen, Proc. SPIE 3358, 54-63, (1998).
44. H.I. Bjelkhagen, Q. Huang, and T.H. Jeong, Progress in color reflection holography, in Sixth Int'l Symposium on Display Holography, ed. by T.H. Jeong, H.I. Bjelkhagen, Proc. SPIE 3358, 104-113 (1998).
45. Y. Gentet, and P. Gentet, "Ultimate" emulsion and its applications: a laboratory-made silver halide emulsion of optimized quality for monochromatic pulsed and full color holography,@ in HOLOGRAPHY 2000, T. H. Jeong, and W. K. Sobotka, eds., Proc. SPIE 4149, 56-62 (2000).
46. T. Kubota, E. Takabayashi, T. Kashiwagi, M. Watanabe, and K. Ueda, Color reflection holography using four recording wavelength, in Practical Holography XV and Holographic Materials VII, ed. by S.A. Benton, S.H. Stevenson, T.J. Trout. Proc. SPIE 4296, 126-133 (2001).
47. D. Kodama, M. Watanabe, and K. Ueda, Mastering process for color graphics arts holograms, in Practical Holography XV and Holographic Materials VII, ed. by S.A. Benton, S.H. Stevenson, T.J. Trout. Proc. SPIE 4296, 198-205 (2001).
48. H.I. Bjelkhagen and D. Vuki… eviƒ : Color holography: a new technique for reproduction of paintings, in Practical Holography XV and Holographic Materials VII, ed. by S.A. Benton, S.H. Stevenson, and T.J. Trout. Proc. SPIE 4659, 83-90 (2002).
49. M. Ulibarrena, L. Caretero, R. Madrigal, S. Blaya, and A. Fimia, Multiple band holographic reflection gratings recorded in new ultra-fine emulsion BBVPan, Opt. Express 11, 3385-3392 (2003).
50. M. Ulibarrena, A new panchromatic silver halide emulsion for recording color holograms, Holographer.org , 1-12 (Jan. 2004).
51. J. Zhu, Y. Zhang, G. Dong, Y. Guo, and L. Guo: Single-layer panchromated gelatin material for Lippmann color holography. Opt. Commun. 241, 17-21 (2004).
52. E. Mirlis, H.I. Bjelkhagen, and M. Turner, Selection of optimum wavelengths for holography recording, in Practical Holography XIX: Materials and Applications, T. H. Jeong, and H. I. Bjelkhagen, eds., Proc. SPIE 5742, 113-118 (2005).
53. Geola, POB 343, Naugarduko 41, LT 2006 Vilnius, Lithuania.
54. SLAVICH Joint Stock Co., Micron Branch Co., 2 pl. Mendeleeva, 152140 Pereslavl-Zalessky, Russia.